|
02 Features
Print To PDF
DisplayTitle Improving Nutrition through Biofortification: Preharvest and Postharvest Technologies
Authors G. Michael Listman,1 Carlos Guzmán,1 Natalia Palacios-Rojas,1 Wolfgang H. Pfeiffer,2 Felix San Vicente,1 and Velu Govindan1
Affiliations 1 International Maize and Wheat Improvement Center, (CIMMYT), Carretera México-Veracruz, Km 45, El Batán, Texcoco, México, 56237.
2 HarvestPlus, Washington, DC, U.S.A.
© 2019 AACC International, Inc.
Abstract
CFWAbstract More than 2 billion people worldwide suffer from “hidden hunger,” wherein they fail to obtain enough nutrients or micronutrients from the foods they eat. Biofortification—the development of micronutrient-dense staple crops using traditional breeding practices and modern biotechnology—is a promising approach to improve nutrition, as part of an integrated, food systems strategy. In work begun in the late 1990s, the CGIAR institutions HarvestPlus, the International Maize and Wheat Improvement Center (CIMMYT), and the International Institute of Tropical Agriculture (IITA), together with numerous national research organizations and scaling partners, have in recent years developed and released in 19 countries in Africa, Asia, and Latin America more than 60 improved varieties of maize and wheat whose grains feature enhanced levels of the essential micronutrients zinc or provitamin A. Use of these varieties is spreading among farmers and consumers, many of whom have diets that lack micronutrients because they cannot afford diverse foods and depend heavily on foods made from staple crops. Eating provitamin A-rich maize has been shown to be as effective as supplementation, and a 2018 study in India found that using zinc-biofortified wheat to prepare traditional foods can significantly improve children’s health.
Trying to reach content?
View Full Article
if you don't have access, become a member
Page Content Micronutrient Malnutrition
After a prolonged decline, as of 2016 global hunger appears to be increasing. In 2017 global hunger affected more than 820 million individuals and was associated predominantly with increased conflict and violence and climate variability, including extreme events such as droughts, heat waves, and destructive storms (9). In addition to hunger itself, more than 2 billion people worldwide suffer from “hidden hunger,” wherein they fail to obtain enough nutrients or micronutrients from the foods they eat to lead healthy, productive lives and, particularly for children, to develop to their full mental and physical potential (14). Micronutrient deficiencies are particularly acute among the poor in developing countries who rely heavily on staple crops (e.g., maize, wheat, rice, etc.) and eat few micronutrient-rich foods such as fruits, vegetables, and animal and fish products. Vitamin A deficiency affects nearly 190 million preschool aged children and 19 million pregnant women, mostly in developing countries, and is directly associated with vision-related disorders, including night blindness, corneal scarring, and blindness (35). Zinc deficiency is also widespread and particularly prevalent in Africa, the eastern Mediterranean, and South and Southeast Asia (34,36). In young children, zinc deficiency increases the risk of diarrheal diseases, pneumonia, malaria, and mortality from these diseases.
The major food cereal crops maize, rice, and wheat, which are grown on hundreds of millions of hectares worldwide and have a combined annual harvest of around 2.5 billion tons of grain (FAOSTAT, www.fao.org/faostat), are vital to diets, cultures, and economies around the world, especially in populous developing regions. Global demand for all three cereals is rising—a trend that is expected to continue through mid-century. Thus, these major cereals offer critical entry points for improving nutrition (25,26). Biofortification, as described here for maize and wheat, is one promising approach to improving nutrition.
Biofortification as a Remediation Approach
Biofortification involves the development of micronutrient-dense staple crops using traditional breeding practices and modern biotechnology (5,15). The approach has multiple advantages when utilized as part of an integrated, food systems strategy to reduce malnutrition and address micronutrient deficiencies:
- It capitalizes on the daily intake of food within households where staple crops predominate, implicitly targeting low-income households.
- Developing biofortified crops is a one-time investment, so recurrent costs are low, relevant germplasm can be shared across borders, and the global coverage of staple crops such as maize and wheat provides a meaningful return on investments.
- Biofortified crop systems are highly sustainable; the varieties developed and released will continue to be grown and consumed year after year, even in the absence of government attention or external funding.
- Breeding for higher levels of provitamin A and zinc in the cereal grain incurs no yield penalty.
Biofortification is just one of several ways micronutrient deficiencies can be of addressed. Other approaches include dietary supplements, fortification of common foods, and more diverse and healthful diets.
Biofortification has been championed by HarvestPlus (www.harvestplus.org), an organization within CGIAR that seeks to improve nutrition and public health by working with various partners to develop and promote biofortified food crops, as well as provide global leadership on biofortification evidence and technology.
There are currently 290 released varieties of 12 different biofortified crops: rice, wheat, maize, cassava, pearl millet, beans, sweet potato, lentils, cowpea, bananas and plantain, sorghum, and Irish potatoes. These varieties have been released in more than 30 countries and tested in more than 60 countries, reaching an estimated 10 million farm households and providing more than 50 million farming household members with access to biofortified foods (13). One example of successful biofortification is the vitamin A-rich, orange-fleshed sweet potato that is grown in numerous countries in Africa. For their development of this crop, researchers from the International Potato Center (CIP) and Howarth Bouis, founder of HarvestPlus, received the 2016 World Food Prize.
Maize and wheat are excellent targets for biofortification because they are widely cultivated, have wide agroecosystem coverage, are important in diets and trade, have useful native genetic variation for improving micronutrient density in the grain, and have a long history as subjects of breeding and genetic research.
Biofortification in Maize and Wheat
A precursor of biofortification of maize was the development of quality protein maize (QPM) by the International Maize and Wheat Improvement Center (CIMMYT) during the 1970s and 1980s. Based on the opaque-2 (o2) maize gene mutation discovered by Purdue University researchers in 1963, QPM features enhanced levels of lysine and tryptophan, which are essential amino acids that can help reduce malnutrition in children (19). The CIMMYT scientists who developed QPM received the 2000 World Food Prize for their work. Despite repeated demonstrations that QPM is more nutritious than conventional maize, village-level evidence that it can improve the health of children who consume a high-maize diet, and proof of its value as a feed for monogastric animals, QPM is not widely grown. Constraints to cultivation include difficulties associated with QPM seed production and the lack of added market value for farmers, because QPM looks like traditional maize and can only be distinguished through laboratory testing (2).
Enhancing Levels of Iron and Zinc in Wheat Grain. In concert with and funded by HarvestPlus, in the late 1990s and early 2000s CIMMYT began searching through the wheat gene pool, particularly among wheat landraces and tetraploid and diploid progenitors of hexaploid wheat, for sources of higher levels of zinc and iron in the grain. The successful integration of novel alleles affecting levels of iron and zinc in the wheat grain from wild relatives of wheat was achieved more recently through use of hexaploid derivatives of crosses between tetraploid Triticum dicoccum and the diploid grass species Aegilops tauschii, as well as directly from T. spelta (21).
High-zinc alleles from these sources were crossed into elite breeding lines, while avoiding or eliminating undesirable genes from wild relatives, and large segregating populations were grown to enable selection for good agronomic traits and disease resistance prior to selecting for zinc. The resulting advanced lines were grown in yield trials in Mexico. Lines that had both a high yield under high-production conditions and featured high levels of zinc in the grain were tested on-station under heat stress, severe and moderate drought stress, and other constraints (31), as well as for desirable processing and end-use quality (11,27,29,30). In parallel with testing in Mexico, the lines were screened at multiple sites in India and Pakistan (32). Genotypes at some locations yielded an additional 12 ppm zinc—the full targeted increment of additional zinc content in the grain (33). The focus on wheat trials in South Asia was due in part to the high incidence of micronutrient deficiencies in the region, the importance of wheat in local diets, and the fact that micronutrients such as iron and zinc are concentrated in the bran of the wheat kernel and consumers in South Asia favor flatbreads, such as chappatis, that are made from milled whole grain. By the same token, the additional iron and zinc contained in biofortified wheat are among the nutrients that are largely lost through milling processes that eliminate the bran.
As a result of this work, six biofortified wheat varieties with zinc contents 6–12 ppm higher than in traditional wheat grains have been released in recent years in Pakistan and India (Fig. 1). These varieties are resistant to wheat stem, stripe, and leaf rust diseases and produce high yields under both optimal conditions and drought or high temperature conditions. One variety was included in fast-track seed multiplication to speed its adoption for cultivation in the Northwestern Plain Zone of India, which is the country’s chief wheat production area. In 2017 a wheat blast-resistant and zinc-enriched wheat variety was released in Bangladesh (6).
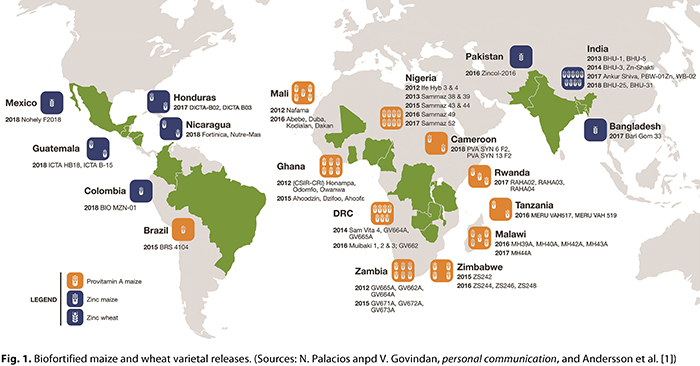
Through dozens of public–private partnerships and farmer participation trials, high-zinc wheat varieties are being tested and promoted in several target regions, including Afghanistan and Nepal, and, in Africa, Ethiopia, Rwanda, and Zimbabwe. CIMMYT shares as many as 200 improved, high-zinc wheat breeding lines with partners in more than 20 developing countries each year. Given the global reach of CIMMYT-derived wheat varieties, which are sown on at least half of the wheat lands cultivated in the developing world, CIMMYT is seeking funding to make high-zinc grain a core trait of all its breeding lines.
Research to develop wheat with higher levels of iron in the grain has progressed, but because the phytic acid in wheat bran more significantly limits the bioavailability of iron, the level of iron needed to overcome phytate binding is much higher than that of zinc, and it has proven difficult to attain through conventional breeding (5).
Maize with High Levels of Zinc in Kernels. Beginning in 2012, CIMMYT and the International Institute of Tropical Agriculture (IITA) began work to develop white grain, zinc-enhanced maize, with public and private partners and funding from HarvestPlus and the CGIAR Research Program on Maize. Through screening of genetically diverse germplasm and use of sources such as QPM varieties (some of which feature high-zinc kernels), as well as ‘ETO Composite’ and the landrace Tuxpeño, breeders have developed many open-pollinated varieties (OPVs) and hybrids with enhanced zinc content in kernels, while maintaining grain yield and other essential traits. In 2018, zinc-enriched maize varieties were released by partners in Colombia (one hybrid), Honduras (two OPVs), and Guatemala (one hybrid and one OPV) (16).
Developing and Releasing Provitamin A-Enhanced Maize. In early screening studies conducted by CIMMYT and IITA, a wide range of provitamin A carotenoid (beta-carotene, beta-cryptoxanthin, and alpha-carotene) levels was found in temperate maize germplasm; later studies with tropical and subtropical maize germplasms showed similar diversity in carotenoid levels. Because tropical maize germplasm contains less beta-carotene than temperate maize germplasm, temperate sources were used initially (17).
In collaboration with HarvestPlus, the Brazilian Agricultural Research Corporation (Embrapa) and other partners, CIMMYT and IITA scientists first crossed provitamin A donors with elite, white maize germplasm that had high yield potential and good agronomic traits, such as disease resistance and drought tolerance (18). F1 crosses then were backcrossed to their respective elite lines. Selection during the first two cycles of inbreeding was primarily for agronomic performance and resistance to lodging and plant and ear diseases. In Zambia, the initial target country for provitamin A-enhanced maize, selected traits included orange color. In many parts of sub-Saharan Africa, not only is white maize preferred for traditional foods, but there is a cultural aversion to yellow maize, which during times of hunger has been imported and distributed in food-relief shipments. Because of its orange color, provitamin A-enhanced maize was perceived by consumers as a different product and was accepted.
Of these new lines, 10–20% with the best traits were tested at multiple locations in target countries to ensure suitability and good performance across environments and growing conditions. Through several subsequent cycles of selection, breeders generated germplasm that was competitive for crucial traits, including good seed production, high grain yield, disease resistance, drought tolerance, food processing quality, and taste. Finally, a second “dose” of high provitamin A germplasm was introduced by crossing lines with novel sources of high provitamin A, followed by further inbreeding and selection (18).
Marker-assisted selection for the desired alleles of key provitamin A genes accelerated genetic gain and allowed breeders to double, sometimes triple, the total concentration of provitamin A in the grain (12,23,37). The breeding target was set at 15 µg of beta-carotene/g (the world average is 0 µg of provitamin A carotenoids/g for white maize and <2 µg/g for yellow maize) to provide an additional 50% of the estimated average requirement for vitamin A in regions where maize is a key food staple.
Maize inbred lines with high levels of provitamin A and desirable agronomic and adaptive traits developed at CIMMYT (for tropical middle altitudes), IITA (for tropical lowlands), and Embrapa were used to generate agronomically competitive hybrids and OPVs featuring high levels of provitamin A in the grain.
Extensive evaluations in collaboration with public and private partners in the Democratic Republic of Congo, Ghana, Mali, Malawi, Nigeria, Tanzania, Zambia, and Zimbabwe led to the selection of promising hybrids and OPVs for further testing in national performance and farmer participation on-farm trials. The testing schemes facilitated the release of more than 40 hybrids and OPVs with up to 90% of the provitamin A breeding target level in eight countries in sub-Saharan Africa and Brazil (1). Breeder seed of the released provitamin A-enhanced maize OPVs and hybrid parental lines has been multiplied and made available to national programs and private seed companies, as well as to community-based seed producers and startup companies, for multiplication, marketing, and other types of distribution.
Positive Effects of Biofortified Maize and Wheat on Nutrition
Nutritional studies have shown that provitamin A-biofortified maize is an efficacious source of vitamin A. Consumption of orange maize has been demonstrated to improve total body vitamin A stores as effectively as supplementation and to significantly improve visual function in marginally vitamin A-deficient children (10,22). Although a bioefficacy study has not yet been conducted, absorption of zinc from eating high-zinc maize has been demonstrated (7). A 2018 study in India found that young children who ate zinc-biofortified wheat in chappatis, puri flatbread, or porridge experienced 17% fewer days with pneumonia and 39% fewer days vomiting than children who consumed conventional wheat products (24).
Use of Advanced Breeding Technologies to Develop Biofortified Maize and Wheat
For more than two decades research teams have applied transgenic techniques to develop biofortified versions of maize, wheat, and other important cereal crops. The earliest and most prominent biofortified crop, Golden Rice, was created in 2000 (38). Despite the fact that Golden Rice and its derivatives are freely licensed and their potential nutritional benefits have been soundly documented, their use on-farm, thus far, has been blocked due to precautionary public health and environmental concerns, as well as strong negative publicity from antibiotechnology interest groups (8).
Despite the challenges encountered in rolling out Golden Rice, crop biofortification research involving transgenics, cisgenics, and other advanced breeding technologies has continued and, among other things, has furthered our understanding of the metabolic pathways associated with micronutrients in cereal grains. Work reported on in 2009 and auguring possible future applications of genetic engineering for biofortification involved the simultaneous modification of the individual metabolic pathways in maize for three vitamins to produce kernels containing 169 times the normal amount of beta-carotene, 6 times the normal amount of ascorbate, and 2 times the normal amount of folate (20). Antioxidant biofortification through transgenic modulation of multiple metabolic pathways, including that for provitamin A in maize, was also reported in 2012 (39).
Given the challenges associated with attaining bioavailable levels of iron in wheat grain through conventional breeding, in 2019 a research team used constitutive expression in bread wheat of the rice nicotianamine synthase 2 (OsNAS2) gene to upregulate biosynthesis of nicotianamine and 2'-deoxymugineic acid, thereby achieving enhanced levels of iron and zinc in the grain (3).
Challenges Facing Biofortified Crops
Three things must happen for biofortification to be successful (15):
- Breeding must be successful—High nutrient density must be combined with high yields and high profitability.
- Efficacy must be demonstrated—The micronutrient status of human subjects must be shown to improve when consuming biofortified varieties as normally eaten as part of the diet. Thus, sufficient nutrients must be retained in processing and cooking, and these nutrients must be sufficiently bioavailable.
- Biofortified crop varieties must be adopted by farmers and the grain consumed by significant numbers of those suffering from micronutrient malnutrition.
Biofortified staple foods can contribute to body stores of micronutrients, such as iron, zinc, and vitamin A, throughout the life cycle, including those of children, adolescents, adults, and the elderly. The potential benefits of biofortification are not equivalent, however, across all of these groups and depend on the amount of a staple food consumed, prevalence of existing micronutrient deficiencies, and micronutrient requirements as affected by daily losses of micronutrients from the body and special needs for processes such as growth, pregnancy, and lactation.
Although nutrient absorption by the body is a prerequisite for preventing micronutrient deficiencies, ultimately changes in the prevalence of micronutrient deficiencies with long-term intake of biofortified staple foods need to be measured directly. Thus, randomized, controlled efficacy trials demonstrating the impact of biofortified crops on micronutrient status at the level of nutrient density thus far achieved (i.e., the minimum target level) are required to provide evidence to support the release of biofortified crops. Another challenge, in the case of provitamin A, is the stability of carotenoids during storage.
Varietal release regulations differ by country and often by states within countries. Typically, for official registry, a variety must be shown to be new, to be distinguishable from other varieties, and to add value in some way. The dissemination strategy for nutrients that are invisible (i.e., iron and zinc) is to “piggyback” them on the genetic resilience and superior agronomic characteristics of elite varieties in specific countries and in this way drive adoption and consumption. For example, high-zinc wheat varieties released in India and Pakistan are resistant to newly evolved wheat rust disease strains in South Asia. Provitamin A carotenoids in maize grain have also been shown to reduce aflatoxin contamination, a serious health concern in sub-Saharan Africa (28).
For nutrients that change the appearance of a crop, as is the case for high-provitamin A maize in Africa, where the most widely consumed maize foods products are made with white grain, nutritional messages must be delivered simultaneously with the release of high-yield, high-profit biofortified varieties to gain the acceptance of farmers and consumers for new orange or yellow varieties.
To realize their full potential, biofortified maize and wheat varieties must be integrated as a core products in research, policy, and food value chains for these crops, which implies that all participants in the value chain, particularly farmers and consumers, must be convinced of their value. Moreover, the dietary benefits of biofortified maize and wheat food products are greater when they are made from whole grains. Research in food science and product development is needed to ensure that whole grain products are accepted by consumers (4).
Acknowledgments
We received funding support from HarvestPlus, including contributions from the United Kingdom Department for International Development (DFID), the Bill & Melinda Gates Foundation, and the CGIAR Research Program on Agriculture for Nutrition and Health (CRP-A4NH). Special thanks are due to national partners who have assisted in testing and promoting biofortified varieties, biofortification teams and colleagues at CIMMYT, IITA, and HarvestPlus, including Meike Andersson, and to the CGIAR Research Programs MAIZE and WHEAT.
G. Michael Listman, a retired communications consultant, worked for 30 years as a science writer and editor and communications specialist at CIMMYT. He has a B.A. degree in journalism from Wayne State University (1979), with a second major in foreign languages and a strong background in science. He has also worked as a reporter and taught English for eight years at the Colegio de Postgraduados, a graduate-level agricultural university in Mexico.
Carlos Guzmán has been working at CIMMYT since 2012, where he now leads the center’s Wheat Chemistry and Quality Laboratory. He is also vice chair of the Expert Working Group on Improving Wheat Quality for Processing and Health of the Wheat Initiative, which aims to advance research on grain quality and apply scientific knowledge to develop nutritious varieties of wheat with desirable grain quality attributes for a wide range of end uses.
Natalia Palacios-Rojas has been a nutritional quality specialist at CIMMYT since 2005. Natalia heads of the center’s Maize Nutritional Quality Laboratory and leads the strategy for maize quality research. She studied microbiology at Andes University in Bogotá, Colombia; conducted her doctoral studies in plant biochemistry at the University of East Anglia and the John Innes Centre, Norwich, England; and had postdoctoral appointments at the University of Dublin and the Max Planck Institute for molecular plant physiology in Potsdam, Germany.
Wolfgang H. Pfeiffer has worked with HarvestPlus for over 12 years, serving as Global Director of Product Development & Commercialization and Plant Breeding Coordinator. Currently, he leads research, development, delivery, and commercialization of biofortified crops in collaboration with over 400 partners in 50 countries. He obtained his M.S. and Ph.D. degrees in Agricultural Sciences from Stuttgart-Hohenheim University in Germany. Prior to joining HarvestPlus, he worked at CIMMYT for more than 20 years as a breeder of bread wheat, durum wheat, and triticale.
Felix San Vicente has lead maize breeding for Latin America at CIMMYT since 2014, leveraging a Ph.D. in plant breeding from Iowa State University and 37 years of experience in quantitative genetics and heterosis relating to tropical maize. With national public and private partners, he has helped develop 22 hybrids and 9 open pollinated varieties grown commercially on 0.5 million hectares in 10 countries.
Velu Govindan is currently a wheat breeder at CIMMYT with more than 14 years of experience in international agriculture and crop improvement. He is developing wheat varieties that feature enhanced levels of grain iron and zinc for improved human nutrition, along with essential core-traits, for South Asia. He completed a Ph.D. in plant breeding at International Crops Research Institute for the Semi-Arid Tropics (ICRISAT), India, and post-doctoral research in Zimbabwe.
References References
- Andersson, M. S., Saltzman, A., Virk, P., and Pfeiffer, W. H. Progress update: Crop development of biofortified staple food crops under HarvestPlus. Afr. J. Food Agric. Nutr. Dev. 17:11905, 2017.
- Atlin, G. N., Palacios, N., Babu, R., Das, B., Twumasi-Afriyie, S., Friesen, D. K., De Groote, H., Vivek, B., and Pixley, K. V. Quality protein maize: Progress and prospects. Plant Breed. Rev. 34:83, 2011.
- Beasley, J. T., Bonneau, J. P., Sánchez-Palacios, J. T., Moreno-Moyano, L. T., Callahan, D. L., Tako, E., Glahn, R. P., Lombi, E., and Johnson, A. A. T. Metabolic engineering of bread wheat improves grain iron concentration and bioavailability. Plant Biotechnol. J. DOI: https://doi.org/10.1111/pbi.13074. 2019.
- Bouis, H., and Saltzman, A. Improving nutrition through biofortification: A review of evidence from HarvestPlus, 2003 through 2016. Global Food Secur. 12:49, 2017.
- Bouis, H. E., Hotz, C., McClafferty, B., Meenakshi, J. V., and Pfeiffer, W. H. Biofortification: A new tool to reduce micronutrient malnutrition. Food Nutr. Bull. 32(Suppl. 1):S31, 2011
- CGIAR Research Program on Wheat. First blast resistant, biofortified wheat variety released in Bangladesh. Published online at https://wheat.org/first-blast-resistant-biofortified-wheat-variety-released-in-bangladesh. CIMMYT, Texcoco, Mexico, 2017.
- Chomba, E., Wescott, C., Wescott, J., Mpabalwani, E., Krebs, N., Patinkin, Z., Palacios-Rojas, N., and Hambidge, M. Zinc absorption from biofortified maize meets the requirements of young rural Zambian children. J. Nutr. 145:514, 2015.
- Dubock, A. An overview of agriculture, nutrition and fortification, supplementation and biofortification: Golden rice as an example for enhancing micronutrient intake. Agric. Food Secur. 6:59, 2017.
- FAO, IFAD, UNICEF, WFP, and WHO. The State of Food Security and Nutrition in the World 2018: Building Climate Resilience for Food Security and Nutrition. Published online at www.fao.org/3/i9553en/i9553en.pdf. Food and Agriculture Organization of the United Nations, Rome, Italy, 2018.
- Gannon, B., Kaliwile, C., Arscott, S., Schmaelzle, S., Chileshe, J., Kalungwana, N., Mosonda, M., Pixley, K., Masi, C., and Tanumihardjo, S. A. Biofortified orange maize is as efficacious as a vitamin A supplement in Zambian children even in the presence of high liver reserves of vitamin A: A community-based, randomized placebo-controlled trial. Am. J. Clin. Nutr. 100:1541, 2014.
- Guzmán, C., Medina-Larqué, A. S., Velu, G., González-Santoyo, H., Singh, R. P., Huerta-Espino, J., Ortiz-Monasterio, I., and Peña, R. J. Use of wheat genetic resources to develop biofortified wheat with enhanced grain zinc and iron concentrations and desirable processing quality. J. Cereal Sci. 60:617, 2014.
- Harjes, C. E., Rocheford, T. R., Bai, L., Brutnell, T. P., Vallabhaneni, R., et al. Natural genetic variation in lycopene epsilon cyclase tapped for maize biofortification. Science 319:330, 2008.
- HarvestPlus. Biofortification: The evidence: A summary of research that supports scaling up of biofortification to improve nutrition and health globally. Published online at https://www.harvestplus.org/evidence-document. HarvestPlus, Washington, DC, 2018.
- HarvestPlus. Nutrition. Published online at www.harvestplus.org/what-we-do/nutrition. HarvestPlus, Washington, DC, 2019.
- International Food Policy Research Institute and Centro Internacional de Agricultura Tropical. Biofortification: Harnessing agricultural technology to improve the health of the poor. Published online at http://ebrary.ifpri.org/utils/getfile/collection/p15738coll2/id/65047/filename/65048.pdf. IFPRI, Washington, DC, 2002.
- Johnson, J. A. Better together: Partnership around zinc maize improves nutrition in Guatemala. Published online at www.cimmyt.org/news/better-together-partnership-around-zinc-maize-improves-nutrition-in-guatemala. CIMMYT, Texcoco, Mexico, 2018.
- Menkir, A., Liu, W., White, W., Maziya-Dixon, B., and Rocheford, T. Carotenoid diversity in tropical-adapted yellow maize inbred lines. Food Chem. 109:521, 2008.
- Menkir, A., Palacios-Rojas, N., Alamu, O., Dias Paes, M. C., Dhliwayo, T., et al. Vitamin A-biofortified maize: Exploiting native genetic variation for nutrient enrichment. Science Brief: Biofortification series, No. 2. M. S. Andersson, H. Bouis, N. Jamora, and M. Major, eds. Published online at www.harvestplus.org/sites/default/files/publications/Vitamin-A-Science-Brief.pdf. CIMMYT, IITA, EMBRAPA, HarvestPlus, and Crop Trust, Bonn, Germany, 2018.
- Mertz, E. T., Bates, L. S., and Nelson, O. E. Mutant gene that changes protein composition and increases lysine content of maize endosperm. Science 145:279, 1964.
- Naqvi, S., Zhu, C., Farre, G., Ramessar, K., Bassie, L., et al. Transgenic multivitamin corn through biofortification of endosperm with three vitamins representing three distinct metabolic pathways. Proc. Natl. Acad. Sci. U.S.A. 106:7762, 2009.
- Ortiz-Monasterio, J. I., Palacios-Rojas, N., Meng, E., Pixley, K., Trethowan, R., and Peña, R. J. Enhancing the mineral and vitamin content of wheat and maize through plant breeding. J. Cereal Sci. 46:293, 2007.
- Palmer, A., Chileshe, J., Hall, A., Barffour, M., Molobeka, N., West, K. P., Jr., and Haskell, M. J. Short-term daily consumption of provitamin A carotenoid biofortified maize has limited impact on breast milk retinol concentrations in Zambia women enrolled in a randomized controlled feeding trial. J. Nutr. 146:1783, 2016.
- Pixley, K., Palacios-Rojas, N., Babu, R., Mutale, R., Surles, R., and Simpungwe, E. Biofortification of maize with provitamin A carotenoids. In: Carotenoids and Human Health. S. Tanumihardjo, ed. Springer, New York, 2013.
- Sazawal, S., Dhingra, U., Dhingra, P., Dutta, A., Deb, S., Kumar, J., Devi, P., and Prakash, A. Efficacy of high zinc biofortified wheat in improvement of micronutrient status, and prevention of morbidity among preschool children and women—A double masked, randomized, controlled trial. Nutr. J. 17:86, 2018.
- Shewry, P. R., and Hey, S. J. The contribution of wheat to human diet and health. Food Energy Sec. 4:178, 2015.
- Shiferaw, B., Prasanna, B. M., Hellin, J., Bänziger, M. Crops that feed the world 6. Past successes and future challenges to the role played by maize in global food security. Food Secur. 3:307, 2011.
- Singh, R. P., and Velu, G. Zinc-biofortified wheat: Harnessing genetic diversity for improved nutritional quality. Science Brief: Biofortification series, No. 1. M. S. Andersson, H. Bouis, and N. Jamora, eds. Published online at www.harvestplus.org/sites/default/files/publications/Science%20Brief-Biofortification-1_Zinc%20Wheat_May2017.pdf. CIMMYT, HarvestPlus, and the Global Crop Diversity Trust, Bonn, Germany, 2017.
- Suwarno, W., Hannok, P., Palacios-Rojas, N., Windham, G., Crossa, J., and Pixley, K. V. Provitamin A carotenoids in grain reduce aflatoxin contamination of maize while combating vitamin A deficiency. Front. Plant Sci. 10:30, 2019.
- Velu, G., Crespo Herrera, L., Guzman, C., Huerta, J., Payne, T., and Singh, R. P. Assessing genetic diversity to breed competitive biofortified wheat with enhanced grain Zn and Fe concentrations. Front. Plant Sci. DOI: https://doi.org/10.3389/fpls.2018.01971. 2019.
- Velu, G., Guzman, C., Mondal, S., Autrique, J. E., Huerta, J., and Singh, R. P. Effect of drought and elevated temperature on grain zinc and iron concentrations in CIMMYT spring wheat. J. Cereal Sci. 69:182, 2016.
- Velu, G., Ortiz-Monasterio, I., Cakmak, I., Hao, Y., and Singh, R. P. Biofortification strategies to increase grain zinc and iron concentrations in wheat. J. Cereal Sci. 59:365, 2014.
- Velu, G., Singh, R., Balasubramaniam, A., Mishra, V. K., Chand, R., Tiwari, C., Joshi, A., Virk, P., Cherian, B., and Pfeiffer, W. Reaching out to farmers with high zinc wheat varieties through public-private partnerships—An experience from Eastern-Gangetic Plains of India. Adv. Food Technol. Nutr. Sci. Open J. 1:73, 2015.
- Velu, G., Singh, R. P., Huerta-Espino, J., Peña-Bautista, R. J., Arun, B., et al. Performance of biofortified spring wheat genotypes in target environments for grain zinc and iron concentrations. Field Crops Res. 137:261, 2012.
- Wessells, K. R., and Brown, K. H. Estimating the global prevalence of zinc deficiency: Results based on zinc availability in national food supplies and the prevalence of stunting. PLoS One. DOI: https://doi.org/10.1371/journal.pone.0050568. 2012.
- WHO. Global Prevalence of Vitamin A Deficiency in Populations at Risk 1995–2005: WHO Database on Vitamin A Deficiency. Published online at https://apps.who.int/iris/bitstream/handle/10665/44110/9789241598019_eng.pdf. World Health Organization, Geneva, Switzerland, 2009.
- WHO. Global and regional trends by UN Regions, 1990–2025. Stunting: 1990–2025. WHO Global Health Observatory Data Repository. Available online at http://apps.who.int/gho/data/node.main.NUTUNREGIONS?lang=en. World Health Organization, Geneva, Switzerland, 2016.
- Yan, J., Kandianis, C. B., Harjes, C. E., Bai, L., Kim, E.-H., et al. Rare genetic variation at Zea mays crtRB1 increases beta-carotene in maize grain. Nat. Genet. 42:322, 2010.
- Ye, X., Klöti, A., Zhang, J., Lucca, P., Beyer, P., and Potrykus, I. Engineering the provitamin A (beta-carotene) biosynthetic pathway into (carotenoid-free) rice endosperm. Science 287:303, 2000.
- Zhu, C., Sanahuja, G., Yuan, D., Farré, G., Arjó, G., et al. Biofortification of plants with altered antioxidant content and composition: Genetic engineering strategies. Plant Biotechnol. J. 11:129, 2013.
|