|
03 Issues & Trends
Print To PDF
DisplayTitle Unconventional Food Plants as an Alternative in Starch Production
Authors José Francisco dos Santos Silveira Junior1 and Alicia de Francisco2
Affiliations Department of Food Science and Technology, Federal University of Santa Catarina, Florianópolis, SC, Brazil
1 E-mail: jjr_silveira@hotmail.com; LinkedIn: https://www.linkedin.com/in/josé-silveira-904a72120; ResearchGate: https://www.researchgate.net/profile/Jose_Junior89; ORCID: https://orcid.org/0000-0003-1576-5674
2 Corresponding author. Department of Food Science and Technology, Cereal Chemistry Laboratory, Federal University of Santa Catarina, Rod. Admar Gonzaga 1346, 88034-000 Florianópolis, SC, Brazil. Tel: +55 48 37215369; E-mail: aliciadf@gmail.com; LinkedIn: https://www.linkedin.com/in/alicia-de-francisco-a802761a; ResearchGate: https://www.researchgate.net/profile/Alicia_De_Francisco; ORCID: https://orcid.org/0000-0001-5862-0657
© 2020 Cereals & Grains Association
Abstract
CFWAbstract The international starch production sector for the food industry is somewhat restricted in the use of chemically modified starches due to country-specific regulations. Therefore, concentrated efforts are needed to identify starch sources with functional characteristics that are similar to chemically modified starches. From this perspective, the potential of five unconventional tropical food plant species is discussed: Canna edulis, Cyperus esculentus, Dioscorea bulbifera, Hedychium coronarium, and Xanthosoma sagittifolium. These tropical food plants can be grown using rustic agronomic management and have high productivity and easily extractable starchy tubers, roots, or rhizomes, which may open possibilities for the substitution of these native starch sources for chemically modified starches in food products.
Trying to reach content?
View Full Article
if you don't have access, become a member
Page Content Starch is the main reserve substance in plants, and it stands out as an abundant, nontoxic, renewable, and low-cost food ingredient. Starch accounts for about 80–90% of all polysaccharides present in human foods (53,72). Billions of dollars are spent annually worldwide on the marketing of starch products that serve a wide range of industrial segments, and starches are a major component in food product applications, as either ingredients or food additives (52).
Corn, potato, wheat, and cassava starches are the most widely used starches in the food industry, serving as thickeners, colloid stabilizers, gelling and volume agents, adhesives, moisture retainers, texturizers, and fat substitutes (60,75). In their native form, applications for starches are restricted because they usually have unwanted functional characteristics. They produce thin, elastic, and cohesive pastes, mainly due to their high hygroscopicity, rapid swelling, loss of viscosity, high tendency to retrograde, low shear strength, and heat treatments (5,7,36).
To overcome these limitations, modification processes are often employed. Chemical, physical, enzymatic, or a combination of these processes are currently employed to obtain customized starch products that meet the requirements for specific food applications (40,53,81).
Chemically modified starches are the most widely used. However, the use of new chemical reagents in starch modification is restricted by country-specific regulations that have increasingly stringent limits related to consumer and environmental protections and occupational safety (35,46,49). As a result, the food industry has been looking for new ways to modify starches or for native starches that have functional characteristics of interest, such as high paste clarity and freeze-thaw stability (35). In this context, both the food industry and farmers are increasingly interested in the identification and development of plants that produce native starches with physicochemical characteristics similar to those of modified commercial starches (30).
Unexplored tubers, roots, rhizomes, bulbs, and corms from tropical plants are emerging as important alternative sources for the starch industry, which could replace chemically modified starches and open new starch markets (7,30). In addition, temperate regions have limitations in their ability to cultivate a large variety of starchy tropical plant species due to climatic and environmental factors, creating the promising potential for growth of these species in tropical regions (2).
Potential of Tropical Biodiversity as a Source for New Starches
Brazil is considered the country with the largest plant biodiversity in the world, with about 55,000 species of plants that represent 22% of the total of approximately 250,000 species that exist in the world (32,61).
Prior to European colonization, South American indigenous populations had a much wider range of food resources than do current populations (2). The hegemonic agricultural model follows a trend in the international market that maintains the stability of the trade balance based on agricultural export monoculture (9).
According to Vilpoux et al. (74), the global human population, which today is approximately 7.3 billion individuals, will exceed 9 billion people by the year 2050. As a result, there is a real need to increase the supply of new starch sources because the production potential of areas dedicated to cereal cultivation will soon not be able to meet demands.
The shortage of food is more critical in the tropics, where most of the world’s population lives (31,78). In times of global climate change, new agricultural production patterns, such as increased production of tropical plant roots and tubers, are required. This is especially true if some countries decide to allocate grain production for biofuel production, or if the cost of energy continues to rise, causing increases in food prices (71).
In general, unconventional tuberous food plants often require only very rough agronomic management and are adaptable to marginal and degraded areas. Cultivation of these plants supports these territories, and although most species belong to different botanical families, they are often found in clusters—a factor that contributes to the diffusion of species (17). In addition, wild species adapt more easily to environmental changes and can be grown in consort with other crops on the same ground, which makes better use of planting areas and increases the availability of organic matter, providing greater soil fertility (17,31).
Although roots and tubers have been used in human foods for millennia, they are also considered foods of the future. They represent alternative raw materials for starch production that can be used to replace cereal grain starches. In addition, they usually have superior nutritional values and can be used for higher quality food applications (66).
Some Starchy Tuberous Cultivars That Deserve Attention
The dietary energy that unexplored starchy plants can provide represents a potential alternative food source in the fight against hunger, especially when considering the paradox of the abundance of unconventional plant species present in regions suffering from starvation (16,71). For a new plant species to be considered suitable for starch exploration on an industrial scale, it is not enough that it is rich in starch—it must also have a good yield, be easy to cultivate, and, especially, be an easily extractable source of starch, such as cassava (74). Five species of unconventional tropical food plants deserve special attention: Canna edulis, Cyperus esculentus, Dioscorea bulbifera, Hedychium coronarium, and Xanthosoma sagittifolium. The chemical composition of their tuberous roots, rhizomes, and bulbs is presented in Table I.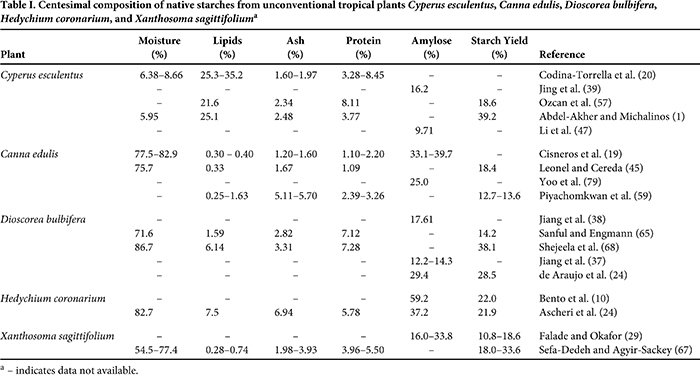 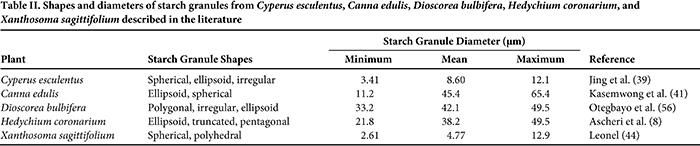
The size and shape of starch granules varies among plant species, and the dimensions of starch granules directly influence the rate of enzymatic digestion and must be considered when choosing potential food applications (61). The shapes and diameter ranges observed for the starches from C. edulis, Cyperus esculentus, D. bulbifera, H. coronarium, and X. sagittifolium are presented in Table II. Preliminary studies (unpublished) have determined the pasting profiles of these starches (Fig. 1).
Dioscorea bulbifera. The family Dioscoreaceae is a very important amylaceous food source found in tropical, subtropical, and temperate regions worldwide. Ninety-five percent of Dioscoreaceae species belong to the genus Dioscorea, commonly known as yam (77). All 600 species of this genus can be grown under highly rustic agronomic management conditions. They do not require periodic seed renewal, are drought tolerant, are undemanding as to soil type, are resistant to pests and diseases, and do not need fertilization or fungicide and insecticide applications, which justifies wide cultivation of these species (27,42).
In general, yams are nutritionally relevant in the human diet, with a high energy value (starch) and high mineral content (K, Na, Mg, Ca, Cu, Zn, P, and Fe) (10). Yams also contain precursors of vitamins A (alpha-carotene, beta-carotene, and retinol), B (thiamine, riboflavin, niacin, and pyridoxine), C (ascorbic acid), and D (ergocalciferol), as well as tannins, steroidal sapogenins, and alkaloids, which in appropriate concentrations may have beneficial health effects (4). The most important species for food and feed applications include D. cayennensis, D. rotundata, D. alata, D. trifida, D. esculenta, and D. bulbifera (77).
Dioscorea spp. also contain undesirable compounds, however, such as alkaloids and cyanogenic glycosides, which when ingested in high quantities or when not eliminated during washing may present toxicity risks (4).
Among the Dioscoreaceae family of plants, the species D. bulbifera deserves special attention because it produces aerial bulbs in which high levels of starch are concentrated (Fig. 2). Although the bulbs are appreciated for their pleasant taste and they have a high nutritional content, they still have low commercial importance (33). In Brazil, D. bulbifera is popularly known as gizzard yam because of the similarity of its aerial tubers to chicken gizzards, but it is also known as butterfly, aerial and rope yams, or carás (77).
D. bulbifera starch has small granules and relatively low levels of amylose, which result in a reduced tendency to retrograde. Some potential food applications include fat substitution to improve mouthfeel in foods such as mayonnaise and salad dressings or in gluten-free pasta (36,54,74).
Canna edulis. C. edulis has potential as a starch source but lacks studies that address possible applications in foods (76). It is a perennial angiosperm in the Cannaceae family, is native to the Andes in South America, and has starch-rich rhizomes (Fig. 3) that have been used as a staple food by indigenous populations for more than 4,000 years (19). Popular names for C. edulis vary according to region and include achira, sagu, arawac, imocoma, chisgua, maraca, and doormat, among others. C. edulis is one of the 25 most consumed starch sources in tropical countries (62).
The cultivation area of C. edulis extends from Mexico to southern Argentina and Brazil, but in Colombia production is more extensive because its starch is popularly used in the production of traditional snacks known as achiras (66,69). C. edulis is also cultivated in other regions, such as Africa, Oceania, and Asia. In countries such as China, Vietnam, Taiwan, and Thailand C. edulis starch is widely used in the food industry for pasta, sauce thickener, condiment, and instant soup applications, as well as in the pharmaceutical industry and biofilm development (23,76).
The cultivation of C. edulis results in high yields because it readily adapts to different climates. It supports different levels of rough agronomic conditions, such as arid, nutrient-poor terrain, but it is often produced on subtropical plateaus at altitudes of 1,000–2,000 m above sea level (48). The production cycle can vary from 10 to 12 months, but it can be harvested after 6–8 months. The rhizome yield can reach 30 t/ha, with starch contents of 70–80 g/100 g of dry rhizome, and its starch is more digestible than other types of starches (62).
The starch granules of C. edulis rhizomes are generally large and high in amylose. They have a high tendency to retrograde and have high resistance to alpha-amylase hydrolysis. It is possible to obtain stable pastes at higher temperatures that are lighter in color and more viscous than cassava starch at the same concentrations. Such characteristics may be of interest for use as a thickener in the food industry, which has sought the same attributes in chemically modified starches (73).
Cyperus esculentus. Cyperus esculentus is another underused plant that has broad production potential. It is a cosmopolitan species that is appreciated for its sweet-flavored tubers (Fig. 4). It is grown in more than 92 countries and is considered one of the most widely distributed plant species (25). Some popular names for Cyperus esculentus include tiririca, bibi, cotufa, tamascal, junça, juncinha, and edible cipero (Brazil); chufa (Spain); earth almond, rush nut, yellow nutsedge, nutgrass, zulu nut, and tiger nut (English-speaking countries) (22,55).
Cyperus esculentus is a perennial grass with high rusticity that is resistant to prolonged periods of drought and is considered an indicator of poor soil quality. It is found in countries with tropical and subtropical climates and in many countries with temperate climates. In the Northern Hemisphere, Cyperus esculentus grows in the southern United States and throughout Europe. The incidence of Cyperus esculentus increases closer to the tropics (3,33,58).
Cyperus esculentus, through the C4 photosynthetic mechanism, is highly efficient in absorbing atmospheric CO2 under water stress, high temperatures, and high light intensity, which results in survival and vegetative persistence. This is why it is considered a weed in several commercial crops, such as sugarcane and cotton (6). Much of the scientific work that deals with the management of Cyperus esculentus highlights alternatives for its control as a weed. The use of Cyperus esculentus as an alternative source of starch is incipient, and there is a lack of work exploring specific extraction conditions to improve processes (34,58,70).
In several countries in the Mediterranean Region, especially Spain, Cyperus esculentus (“earth almonds”) is appreciated for its almond flavor. It is used to make a refreshing, nonalcoholic milky-colored beverage (water-soluble extract) called horchata de chufa (63). Horchata de chufa, originally made by Muslims, has been a traditional Spanish product with notable economic importance for several centuries. It represents perhaps the most cost-effective application of Cyperus esculentus in the food industry (51,55,72). Each summer in Spain approximately 50 million liters of horchata de chufa are sold, resulting in annual revenues of approximately 60 million euros. To meet this demand, around 1,200 ha of Cyperus esculentus are planted and, on average, 10 t/ha are harvested (50,63). Horchata de chufa is most commonly found in the Valencia region, but its consumption has expanded to the United Kingdom, France, and Portugal, as well as to the United States and Argentina (33,50). Some products derived from horchata de chufa include chocolates, jellies, beers, and liqueurs. It is also used as an alternative to cow’s milk in fermented products and yogurts in African countries (66).
Hedychium coronarium. H. coronarium is an unconventional crop with high potential for industrial starch extraction. It has high rusticity and an unrestrained dissemination capacity (11). It is found in tropical Asian regions ranging from the Himalayas to China. In the Americas, it is found from the coastal regions of the United States to Argentina and Brazil, where it is popularly known as the marsh lily (77).
In addition to its high starch content, the proliferation capacity of H. coronarium represents another strong reason for the exploitation of this species. Because it is considered an invasive crop in agricultural regions and among native vegetation, much of the efforts of the agrobusiness sector, as well as the scientific community, are focused on controlling the species (21,26).
H. coronarium rhizomes contain a wide variety of chemical compounds that are used in folk medicine (Fig. 5). Improvement of essential oil extraction techniques raises the possibility of developing functional and nutraceutical foods. Some of the chemical compounds present in the rhizomes have antibacterial, antifungal, and insecticidal properties, which can serve as a basis for formulation of natural preservatives applicable for food products (12,64). Starch extracted from H. coronarium rhizomes has chemical, structural and thermal properties that could be suitable for food industry applications, such as high amylose content, low crystallinity, and pastes with high viscosity and a tendency to retrograde (11).
To date, the economic exploitation of H. coronarium is mostly related to floriculture, and it is incipient for the manufacture of paper and derivatives from the aerial vegetative parts of the plant (17). There are no published papers addressing the application of H. coronarium starch in food formulations.
Xanthosoma sagittifolium. Among the cultivars discussed in this article, X. sagittifolium could be considered the more important alternative energy source in the traditional diet of tropical regions of the Atlantic coast (13). X. sagittifolium is native to the southern region of the Americas but is widespread and well adapted in tropical areas of Africa and Asia as well. It is recognized by the popular names taioba, taió, tânia, taoiá, tanier, yatuia, cocoyam, and malanga, among others (13,22).
A member of the Araceae family, which includes approximately 109 genera and 4,000 species, X. sagittifolium is a perennial, robust, monocotyledonous leafy species with bulky stems and tubers (Fig. 6) that have the highest starch concentration among edible aracean plants (18).
The agricultural yield of X. sagittifolium tubers is approximately 30 t/ha, with an average yield of 20% starch (in relation to the initial tuber weight). This yield represents starch production potential that could reach up to approximately 10 t/ha (14,16).
The food uses of X. sagittifolium include cooked tubers incorporated in traditional recipes for immediate consumption, as well as production of flour for breadmaking. Starch extraction provides an alternative to increase shelf life (15,22,80). Although X. sagittifolium tubers also contain calcium oxalate, an undesirable chemical compound, the compound can be eliminated by continuous rinsing or during the cooking process, where it remains in the water, rendering the food safe for consumption (28,43,67).
X. sagittifolium starch is a promising alternative to conventional commercial starches from sources such as corn, wheat, potatoes, and cassava due to the high starch yield from tubers and a high starch extraction yield. The starch granules of this cultivar are predominantly small, which favors application in formulating ready-made sauces (80).
Conclusions
The unexplored potential of the plant species described in this article justifies the need for studies to determine the physicochemical nature, extraction processes, characterization, modification, and application of starches from these unconventional food plants. The extraction of unconventional starches may provide an alternative for applications where specific functional characteristics are required and provide and opportunity to replace chemically modified starches with eco-friendly native sources. This, in turn, may increase income for developing countries in subtropical and tropical regions and contribute to the economic security of these regions.
José Francisco dos Santos Silveira Junior is a food technologist at the Federal Technological University of Paraná (UTFPR – Brazil) and holds a master’s degree in food science from the Federal University of Santa Catarina (UFSC – Brazil). He is currently part of the Cereal Science Laboratory (LAB CERES) as a doctoral student in food science (UFSC), under the guidance of Dr. Alicia de Francisco, with the theme “Characterization of Starches Extracted from Unconventional Food Plants (PANCs) and Its Relationship to Modified Cassava Starches.”
Alicia de Francisco is currently a major professor in the Food Science and Technology Department of the Federal University of Santa Catarina (UFSC) in the area of cereal science and technology and food microscopy. Alicia joined the UFSC in 1993. Prior to that, she worked as a researcher at the Carlsberg Research Center in Denmark after working for 7 years with P&D at the Quaker Oats Company in Illinois. Alicia’s main research interest is in dietary fibers, beta-glucans, whole grains, gluten, and nonconventional plants as sources of starch. She is the author of more than 100 refereed papers and more than 100 conference abstracts and presentations. Alicia is a Kansas State University graduate and has been an active member of the Cereals & Grains Association for more than 30 years.
References References
- Abdel‐Akher, M., and Michalinos, A. N. Separation and purification of starch from chufa nut tubers (Cyperus esculentus). Stärke 15:329, 1963.
- Aceituno, F. J., and Loaiza, N. The origins and early development of plant food production and farming in Colombian tropical forests. J. Anthropol. Archaeol. 49:161, 2018.
- Adebayo-Oyetoro, A. O., Ogundipe, O. O., Adeyeye, S. A. O., Akande, E. A., and Akinyele, A. B. Production and evaluation of tiger nut (Cyperus esculentus) milk flavoured with Moringa oleifera leaf extract. Curr. Res. Nutr. Food. Sci. 7:265, 2019.
- Adebowale, A. R. A., Wahab, A. B., Sobukola, P. O., Obadina, A. O., Kajihausa, E. O., Adegunwa, O. M., Sanni, O. L., and Tomlins, K. The antinutritional and vitamin composition of high‐quality yam flour as affected by yam species, pretreatment, and drying method. Food. Sci. Nutr. 6:1985, 2018.
- Ai, Y., and Jane, J. L. Gelatinization and rheological properties of starch. Starch/Stärke 67:213, 2015.
- Ajao, A. A., Oladipo, O. A., and Saheed, S. A. A review on the ambit and prospects of C3 and C4 plants in Nigeria. Sci. Cold Arid. Reg. 9:0587, 2017.
- Alcázar-Alay, S. C., and Meireles, M. A. A. Propriedades físico-químicas, modificações e aplicações de amidos de diferentes fontes botânicas. Ciênc. Tecnol. Aliment. 35:215, 2015.
- Ascheri, D. P. R., Moura, W. D. S., Ascheri, J. L. R., and De Carvalho, C. W. P. Caracterização física e físico-química de rizomas e amido do lírio-do-brejo (Hedychium coronarium). Pesqui. Agropecu. Trop. 40:159, 2010.
- Bais-Moleman, A. L., Schulp, C. J., and Verburg, P. H. Assessing the environmental impacts of production- and consumption-side measures in sustainable agriculture intensification in the European Union. Geoderma 338:555, 2019.
- Bekele, A., and Bekele, E. Proximate and mineral composition variability in Ethiopian yam (Dioscorea spp.). J. Food. Nutr. Sci. 6:12, 2018.
- Bento, J. A. C., Ferreira, K. C., de Oliveira, A. L. M., Lião, L. M., Caliari, M., and Júnior, M. S. S. Extraction, characterization and technological properties of white garland-lily starch. Int. J. Biol. Macromol. 135:422, 2019.
- Bernardo, C. O., Ascheri, J. L. R., Chávez, D. W. H., and Carvalho, C. W. P. Ultrasound assisted extraction of yam (Dioscorea bulbifera) starch: Effect on morphology and functional properties. Starch/Stärke. DOI: https://doi.org/10.1002/star.201700185. 2018.
- Boakye, A. A., Wireko‐Manu, F. D., Oduro, I., Ellis, W. O., Gudjónsdóttir, M., and Chronakis, I. S. Utilizing cocoyam (Xanthosoma sagittifolium) for food and nutrition security: A review. Food. Sci. Nutr. 6:703, 2018.
- Brockman, H. Renewable Chemicals and Bioproducts: A Potential for Agricultural Diversification and Economic Development. Bull. 4875. Published online. Department of Agriculture and Food, South Perth, WA, Australia, 2016.
- Calle, J., Benavent‐Gil, Y., Garzón, R., and Rosell, C. M. Exploring the functionality of starches from corms and cormels of Xanthosoma sagittifolium. Int. J. Food Sci. Technol. 54:2494, 2019.
- Casas, A., Ladio, A. H., and Clement, C. R. Ecology and evolution of plants under domestication in the neotropics. Front. Ecol. Evol. 7:231, 2019.
- Chan, E. W. C., and Wong, S. K. Phytochemistry and pharmacology of ornamental gingers, Hedychium coronarium and Alpinia purpurata: A review. J. Integr. Med. 13:368, 2015.
- Chandrasekara, A., and Kumar, T. J. Roots and tuber crops as functional foods: A review on phytochemical constituents and their potential health benefits. Int. J. Food Sci. DOI: https://doi.org/10.1155/2016/3631647. 2016.
- Cisneros, F. H., Zevillanos, R., and Cisneros-Zevallos, L. Characterization of starch from two ecotypes of Andean achira roots (Canna edulis). J. Agric. Food Chem. 57:7363, 2009.
- Codina-Torrella, I., Guamis, B., and Trujillo, A. J. Characterization and comparison of tiger nuts (Cyperus esculentus L.) from different geographical origin: Physico-chemical characteristics and protein fractionation. Ind. Crops Prod. 65:406, 2015.
- Costa, R. O., Batisteli, A. F., Espindola, E. L. G., and Silva Matos, D. M. Invasive Hedychium coronarium inhibits native seedling growth through belowground competition. Flora. DOI: https://doi.org/10.1016/j.flora.2019.151479. 2019.
- Croat, T. B., Delannay, X., and Ortiz, O. O. A revision of Xanthosoma (Araceae). Part 2. Central America. Aroideana 40:504, 2017.
- Da Costa, J. D. J. G., De Freitas, K. M. L., Santos, F. F., Montagner, T. L., Sant’Ana, G. C. F., Amaral, P. F. F., and Leão, M. H. M. R. Extração do amido do resíduo de junça (Cyperus esculentus): Otimização e caracterização. Acta Tecnol. 13:121, 2018.
- de Araujo, V. C., Rincão, A. M., and Padilla, F. Caracterização do amido nativo de Dioscorea bulbifera. Arch. Latinoam. Nutr. 54:241, 2004.
- De Castro, O., Gargiulo, R., Del Guacchio, E., Caputo, P., and De Luca, P. A molecular survey concerning the origin of Cyperus esculentus (Cyperaceae, Poales): Two sides of the same coin (weed vs. crop). Ann. Bot. 115:733, 2015.
- De Castro, W. C., Almeida, R. V., Leite, M. B., Marrs, R. H., and Matos, D. S. Invasion strategies of the white ginger lily Hedychium coronarium J. König (Zingiberaceae) under different competitive and environmental conditions. Environ. Exp. Bot. 127:55, 2016.
- Degefa, I., and Anbessa, B. Traditional agronomic practices of yam (Dioscorea species) in Abaya Woreda, Southern Ethiopia. Nat. Sci. 5:499, 2017.
- De Lima, A. S. G., and Krupek, R. A. Caracterização morfológica, anatômica, e toxinas endógenas em Colocasia esculenta e Xanthosoma sagittifolium. Luminária 18:01, 2016.
- Falade, K. O., and Okafor, C. A. Physicochemical properties of five cocoyam (Colocasia esculenta and Xanthosoma sagittifolium) starches. Food Hydrocoll. 30:173, 2013.
- Fan, X., Zhang, S., Lin, L., Zhao, L., Liu, A., and Wei, C. Properties of new starches from tubers of Arisaema elephas, yunnanense and erubescens. Food Hydrocoll. 61:183, 2016.
- Farrukh, M. U., Bashir, M. K., and Rola-Rubzen, F. Exploring the sustainable food security approach in relation to agricultural and multi-sectoral interventions: A review of cross-disciplinary perspectives. Geoforum 108:23, 2020.
- Fernandes, G. W., Vale, M. M., Overbeck, G. E., Bustamante, M. M. C., Grelle, C. E. V., et al. Dismantling Brazil’s science threatens global biodiversity heritage. Perspect. Ecol. Conserv. 15:239, 2017.
- Follak, S., Aldrian, U., Moser, D., and Essl, F. Reconstructing the invasion of Cyperus esculentus in Central Europe. Weed Res. 55:289, 2015.
- Ghosh, A., Mondal, D., Bera, S., Poddar, R., Kumar, A., Bandopadhyay, P., and Ghosh, R. K. Halosulfuron methyl: For effective control of Cyperus spp. in sugarcane (Saccharum officinarum L.) and its residual effect on succeeding green-gram (Vigna radiata L.). J. Crop Weed 13:167, 2017.
- Greer, F. R. Use of starch and modified starches in infant feeding: A historical perspective. J. Pediatr. Gastroenterol. Nutr. 66:30, 2018.
- Hornung, P. S., Avila, S., Lazzarotto, M., Da Silveira Lazzarotto, S. R., de Siqueira, G. L. D. A., Schnitzler, E., and Ribani, R. H. Enhancement of the functional properties of Dioscoreaceas native starches: Mixture as a green modification process. Thermochim. Acta 649:31, 2017.
- Jiang, Q., Gao, W., Li, X., Wang, H., Xia, Y., and Xiao, P. Comparison of starches separated from different Dioscorea bulbifera Linn. cultivars. Starch/Stärke 64:531, 2012.
- Jiang, Q., Gao, W., Li, X., Xia, Y., Wang, H., Wu, S., Huang, L., Liu, C., and Xiao, P. Characterizations of starches isolated from five different Dioscorea L. species. Food Hydrocoll. 29:35, 2012.
- Jing, S., Yan, X., Ouyang, W., Xiang, H., and Ren, Z. Study on properties of Cyperus esculentus starch grown in Xinjiang, China. Starch/Stärke 64:581, 2012.
- Karaki, N., Aljawish, A., Humeau, C., Muniglia, L., and Jasniewski, J. Enzymatic modification of polysaccharides: Mechanisms, properties, and potential applications: A review. Enzyme Microb. Technol. 90:1, 2016.
- Kasemwong, K., Piyachomkwan, K., Wansuksri, R., and Sriroth, K. Granule sizes of canna (Canna edulis) starches and their reactivity toward hydration, enzyme hydrolysis and chemical substitution. Starch/Stärke 60:624, 2008.
- Kouam, E. B., Avana-Tientcheu, M. L., Lekeumo, V. D., Akitio, H. M., Khasa, D. P., and Pasquet, R. S. Agro-ecological distribution of the phenotypic diversity of aerial yam (Dioscorea bulbifera L.) in Cameroon using multivariate analysis: Prospect for germplasm conservation and improvement. Open Agric. 3:190, 2018.
- Kumar, A., Patel, A. A., and Gupta, V. K. Reduction in oxalate, acridity, phenolic content and antioxidant activity of Amorphophallus paeoniifolius var. Gajendra upon cooking. Int. Food Res. J. 24:1614, 2017.
- Leonel, M. Análise da forma e tamanho de grânulos de amidos de diferentes fontes botânicas. Food Sci. Technol. 27:579, 2007.
- Leonel, M., and Cereda, M. P. Caracterização físico-química de algumas tuberosas amiláceas. Food Sci. Technol. 22:65, 2002.
- Lewicka, K., Siemion, P., and Kurcok, P. Chemical modifications of starch: Microwave effect. Int. J. Polym. Sci. DOI: https://doi.org/10.1155/2015/867697. 2015.
- Li, X., Fu, J., Wang, Y., Ma, F., and Li, D. Preparation of low digestible and viscoelastic tigernut (Cyperus esculentus) starch by Bacillus acidopullulyticus pullulanase. Int. J. Biol. Macromol. 102:651, 2017.
- Lobo-Arias, M., Medina-Cano, C. I., Grisales-Arias, J. D., Yepes-Agudelo, A. F., and Álvarez-Guzmán, J. A. Caracterización y evaluación morfológicas de la colección colombiana de achira, Canna edulis Ker Gawl.(Cannaceae). Cienc. Tecnol. Agropecuária 18:47, 2017.
- Luallen, T. Utilizing starches in product development. Page 545 in: Starch in Food: Structure, Function and Applications, 2nd ed. M. Sjöö and L. Nilsson, eds. Woodhead Publishing, Duxford, U.K., 2018.
- Maduka, N., and Ire, F. S. Tigernut plant and useful application of tigernut tubers (Cyperus esculentus)—A review. J. Appl. Sci. Technol. 18:1, 2018.
- Martín-Esparza, E., and González-Martínez, C. Horchata de chufa: A traditional Spanish beverage with exceptional organoleptic, nutritive, and functional attributes. Page 371 in: Functional Properties of Traditional Foods. K. Kristbergsson and S. Ötles, eds. Springer Nature US, New York, NY, 2016.
- Martinez, E. L., and Fernandez, F. J. B. Economics of production, marketing and utilization. Page 87 in: Corn: Chemistry and Technology, 3rd ed. S. O. Serna-Saldivar, ed. Cereals & Grains Association, St. Paul, MN, 2019.
- Masina, N., Choonara, Y. E., Kumar, P., Du Toit, L. C., Govender, M., Indermun, S., and Pillay, V. A review of the chemical modification techniques of starch. Carbohydr. Polym. 157:1226, 2017.
- Mingle, E., Sanful, R. E., and Engmann, F. N. Sensory and physicochemical properties of bread made from aerial yam (Dioscorea bulbifera) and wheat (Triticum aestivum) flour. Int. J. Innov. Food. Sci. Technol. 5:5, 2017.
- Mohdaly, A. A. R. A. A. Tiger nut (Cyperus esculentus L.) oil. Page 243 in: Fruit Oils: Chemistry and Functionality. Ramadan, M. F., ed. Springer Nature Switzerland AG, Basel, Switzerland, 2019.
- Otegbayo, B., Oguniyan, D., and Akinwumi, O. Physicochemical and functional characterization of yam starch for potential industrial applications. Starch/Stärke 66:235, 2014.
- Ozcan, M. M., Gumuscu, A., Er, F., Arslan, D., and Ozkalp, B. Chemical and fatty acid composition of Cyperus esculentus. Chem. Nat. Compd. 46:276, 2010.
- Pitrel, B., Boutteaux, P., and Davy, M. Biology and infestation of yellow nutsedge (Cyperus esculentus) in vegetable crops—Research of means of control. Page 38 in: 23e Conférence du COLUMA. Journées Internationales sur la Lutte contre les Mauvaises Herbes, Dijon, France. Association Française de Protection des Plantes, Alfort, France, 2016.
- Piyachomkwan, K., Chotineeranat, S., Kijkhunasatian, C., Tonwitowat, R., Prammanee, S., Oates, C. G., and Sriroth, K. Edible canna (Canna edulis) as a complementary starch source to cassava for the starch industry. Ind. Crops Prod. 16:11, 2002.
- Pullaiah, T., Bahadur, B., and Krishnamurthy, K. V. Plant biodiversity. Page 177 in: Plant Biology and Biotechnology. Vol 1: Plant Diversity, Organization, Function and Improvement. B. Bahadur, M. V. Rajam, L. Sahijram, and K. V. Krishnamurthy, eds. Springer Nature India, New Delhi, India, 2015.
- Qi, X., and Tester, R. F. Effect of native starch granule size on susceptibility to amylase hydrolysis. Starch/Stärke 68:807, 2016.
- Reddy, P. P. Achira, Canna edulis. Page 281 in: Plant protection in tropical root and tuber crops, Springer Nature India, New Delhi, India, 2015.
- Roselló-Soto, E., Barba, F., Putnik, P., Bursać Kovačević, D., Lorenzo, J., and Cantavella-Ferrero, Y. Enhancing bioactive antioxidants’ extraction from “Horchata de Chufa” by-products. Foods 7:161, 2018.
- Sakhanokho, H. F., and Rajasekaran, K. Hedychium essential oils: Composition and uses. Page 49 in: Essential Oil Research. S. Malik, ed. Springer Nature Switzerland AG, Cham, Switzerland, 2019.
- Sanful, R. E., and Engmann, F. N. Physico-chemical and pasting characteristics of flour and starch from aerial yam. Am. J. Food. Sci. Nutr. 3:1, 2016.
- Saranraj, P., Behera, S. S., and Ray, R. C. Traditional foods from tropical root and tuber crops: Innovations and challenges. Page 159 in: Innovations in Traditional Foods, 1st ed. C. M. Galanakis, ed. Woodhead Publishing, Duxford, U.K., 2019.
- Sefa-Dedeh, S., and Agyir-Sackey, E. K. Chemical composition and the effect of processing on oxalate content of cocoyam Xanthosoma sagittifolium and Colocasia esculenta cormels. Food Chem. 85:479, 2004.
- Shejeela, P. S., Mohan, V. R., Jesuda, L. L., and Soris, P. T. Nutritional and antinutritional evaluation of wild yam (Dioscorea spp.). Trop. Subtrop. Agroecosyst. 14:723, 2011.
- Simi, M. C., Aneena, E. R., Panjikkaran, S. T., Sharon, C. L., and Sheela, K. B. Standardisation and quality evaluation of Queensland arrowroot (Canna edulis L.) based custard powder. J. Trop. Agric. 54:35, 2016.
- Soltani, N., Shropshire, C., and Sikkema, P. H. Yellow nutsedge (Cyperus esculentus L.) control in corn with various rates of halosulfuron. Can. J. Plant. Sci. 98:628, 2018.
- Stephen, A. M., and Phillips, G. O., eds. Food Polysaccharides and Their Applications, 2nd ed. CRC Press, Boca Raton, FL, 2006.
- Taylor, M., Lebot, V., McGregor, A., and Redden, R. J. Sustainable production of roots and tuber crops for food security under climate change. Page 359 in: Food Security and Climate Change. S. S. Yadav, R. J. Redden, J. L. Hatfield, A. W. Ebert, and D. Hunter, eds. John Wiley & Sons, Hoboken, NJ, 2019.
- Vankar, P. S., and Srivastava, J. A review—Canna the wonder plant. J. Text. Eng. Fashion Technol. 4:159, 2018.
- Vilpoux, O. F., Brito, V. H., and Cereda, M. P. Starch extracted from corms, roots, rhizomes, and tubers for food application. Page 103 in: Starches for Food Application: Chemical, Technological and Health Properties. M. T. Pedrosa Silva Clerici and M. Schmiele, eds. Academic Press, London, U.K., 2019.
- Waterschoot, J., Gomand, S. V., Fierens, E., and Delcour, J. A. Starch blends and their physicochemical properties. Starch/Stärke 67:14, 2015.
- Xie, F., Gong, S., Zhang, W., Wu, J., and Wang, Z. Potential of lignin from Canna edulis Ker residue in the inhibition of alpha-D-glucosidase: Kinetics and interaction mechanism merging with docking simulation. Int. J. Biol. Macromol. 95:592, 2017.
- Xu, Z., and Chang, L., eds. Identification and Control of Common Weeds: Volume 3. Springer Nature Singapore, Singapore, 2017.
- Yadav, S. S., Redden, R. J., Hatfield, J. L., Ebert, A. W., and Hunter, D., eds. Food Security and Climate Change. John Wiley & Sons, Hoboken, NJ, 2019.
- Yoo, S.-H., Perera, C., She, J., Ye, L., Suh, D. S., and Jane, J. L. Molecular structure of selected tuber and root starches and effect of amylopectin structure on their physical properties. J. Agric. Food Chem. 57:1556, 2009.
- Zhu, F. Structure, properties, and applications of aroid starch. Food Hydrocoll. 52:378, 2016.
- Zia-ud-Din, Xiong, H., and Fei, P. Physical and chemical modification of starches: A review. Crit. Rev. Food. Sci. Nutr. 57:2691, 2017.
|